Evaluation of GLA-GCM upper-tropospheric moisture using TOVS radiance
observations
NASA Goddard Laboratory for Atmospheres Greenbelt, Maryland
This document is abreviated from Salathé, E. P., D. Chesters,
and Y. C. Sud, 1995: Evaluation of the upper-tropospheric moisture
climatology in a general circulation model using TOVS radiance observations,
J. Clim., 8, 2404-2414.
1. Introduction
General circulation models (GCMs) are valuable devices for
addressing global change issues. However, a GCM and its physical
processes need to be evaluated for the realism of the simulated
atmospheric dynamics and thermodynamics. In particular, accurate
simulation of the vertical moisture profiles is central to the accurate
modeling of the diabatic heating of the atmosphere by radiative and
condensation heating processes. For example, the accuracy of
simulated global warming, in response to increased greenhouse
gases in the atmosphere, crucially depends upon the influence of
water vapor and clouds. Therefore, a model projecting such
scenarios must predict water vapor and clouds consistent with
observations. In this paper, we use TOVS radiance data to evaluate
the ability of the GLA model to depict the climatology of upper
tropospheric moisture and its interannual variability. We also
examine the effect of including downdrafts in the convective
parameterization on the simulated moisture.
2. TOVS Data
TOVS radiance data are archived by NOAA as idealized nadir-
viewing clear-sky brightness temperatures after applying corrections
to the raw observations for satellite view angle and cloud
contamination (see Wu et al. ,1993 for details). The TOVS data set
used in this study is described by Chesters and Sharma (1992). The
brightness temperatures presented here are the linear combination of
the TOVS 6.7 and 7.2 µm channels used to enhance the sensitivity
to the upper-most levels of the water vapor profile. To create daily
images, the data along the satellite sub-orbital swaths were placed on
a polar stereographic grid using the NOAA objective analysis, and
then interpolated to a 5°x5° rectangular grid from 40S to 40N. 6%
of the daily images were unrecoverable or discarded due to
excessive missing observations; Seasonal averages are obtained
from the remaining data
3. The GLA GCM
The version of the Goddard Laboratory for Atmospheres (GLA)
GCM has a coarse resolution (4° x 5° x 17-layers) and is fully
documented in Sud and Walker (1993, Table 3). The model is
driven by observed SST. The simulated fields are interpolated to
the 5x5 degree grid of the TOVS data, and daily images of TOVS-
like radiances are computed from the temperature and moisture
profiles using a narrow band radiation model (Salathé and Smith,
1994).
4. Results
4.1. Climatology
To compare the climatological seasonal cycle of brightness
temperature as observed by TOVS and simulated by the GLA-GCM,
seasonal averages were taken over the 10 year period 1979-1988.
The correspondence between the observed (Fig 1) and computed
(Fig 2) fields is generally quite good; the GCM captures most of the
main regional features and their seasonal cycle. The brightness
temperatures computed from the GCM are on average 2.8 K higher
than observed. This bias is consistent with the bias found by
Salathé and Smith (1994) in comparing brightness temperatures
computed from precisely measured atmospheric soundings to GOES
observations. The difference between the model and observations
tends to be largest where the observed brightness temperatures are
close to maximum or minimum. The GLA-GCM does not produce
as strong a contrast between moist and dry regions as is observed.
This effect is most pronounced JJA. The GCM indicates a dryer
upper troposphere than the observations over the moist convective
regions (e.g. over Central America and along the equator) and a
moister upper troposphere in the dry subtropical subsidence regions
just north and south of the equator (e.g. over the Arabia)

Fig 1. Seasonal cycle of observed brightness temperatures in K.
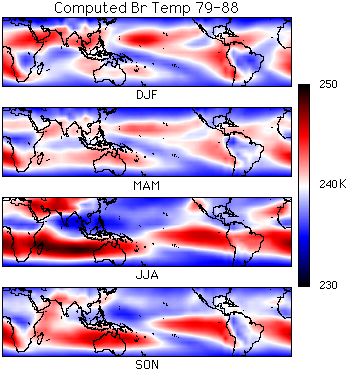
Fig 2. Seasonal cycle of computed brightness temperatures in K.
The region of high brightness temperatures across the southern
Indian Ocean in JJA is an exception to the trend of the model¹s
inability to capture extreme conditions well. In the observations,
this region exhibits lower values than are found over N. Africa and
the Middle East and comparable values to those in the East Pacific.
In the GCM, this region gives greater brightness temperatures than
are found over the Middle East or the East Pacific. In JJA, the
contrast between the high values over the S. Indian ocean and the
low values in the moist convective region over the bay of Bengal are
similar for both the model and the observations. The gradient is
weaker in the model, as the model becomes too dry in the middle of
the Indian Ocean.
The linear correlations of the spatial patterns of 10 year monthly
average brightness temperature are nearly uniform throughout the
seasonal cycle, varying from 82% in June to 78% in December.
The correlation of year-by-year monthly averages of computed and
observed brightness temperatures over the 10 year period is shown in
Fig 3 (top line). The correlations are high during northern summer
(JJA), but low during DJF. An exception is the beginning of 1983
during the El Niño, when the correlation is high throughout the
year. The statistical significance of the lowest correlations is
marginal, the highest values (80%) are significant. Thus the model
can capture the patterns observed in JJA of individual years, but not
those of individual DJF months. The 10 year model climatology for
DJF months, however, does correspond well to the observed
climatology. In JJA, convective centers are strongly centered on the
N. Hemisphere land masses, whereas in DJF the convection is more
sensitive to SST anomalies. Thus the weaker correlation in DJF
may be attributable to the SST anomalies only weakly influencing
the upper tropospheric moisture in the GCM.
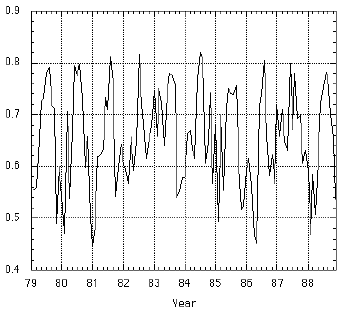
Fig 3. Correlation of monthly brightness temperature patterns.
Upper solid line: Observed and computed brightness temperatures.
Lower solid line: Same but with seasonal cycle removed. Dashed
line: Same as solid but using simulation with downdrafts.
4.2. Interannual Variability
The ability of the GLA model to capture the interannual
variability in upper tropospheric moisture as indicated by the TOVS
data can be examined by comparing their departures from the
seasonal cycles. The monthly mean computed and observed
brightness temperature anomaly fields are uncorrelated for most of
the 10 year period (Fig 3 lower line). The correlations may be
significant only for the1983 El Niño, indicating that the model is
sensitive to this large SST anomaly. Otherwise, the GCM does not
capture the interannual variability of upper tropospheric moisture.
4.3. Convective-scale Downdrafts
The convective parameterization used in the GLA GCM for the
simulation discussed above does not include the influence of
convective scale downdrafts. Sud and Walker (1993) recently
introduced in the convective parameterization a new mass-flux
parameterization for downdrafts that brings colder and drier air near
the surface, displacing the warm and moist air upwards, and thereby
moistening upper levels. The scheme was found to produce
significant changes in the tropical precipitation and even the Hadley
circulation (Sud and Walker, 1993). To evaluate the effect of the
downdrafts on upper-tropospheric moisture in the model, we
compare the brightness temperatures computed from a 1979-1980
run of the GLA GCM to the TOVS data. Including downdrafts has
no significantly significant effect on the upper tropospheric
moisture.
5. Conclusion
This study not only shows the GLA-CGM¹s strengths and
deficiencies, but it gives us confidence in the value of the TOVS data
for model evaluation and validation.
References
- Chesters, D. and O. Sharma, 1992: An Atlas of Upper
Tropospheric Radiances Observed in the 6 to 7 Micrometer Water
Vapor Band Using TOVS Data from the NOAA Weather Satellites
During 1979-1991. NASA Technical Memo 104563, Goddard
Space Flight Center, Greenbelt, MD., 183pp.
- Salathé, E. P. and R. B. Smith, 1994: Comparison of 6.7
micron radiances computed from aircraft soundings and observed
from GOES-VAS. J. Geophys. Res., Submitted
- Sud, Y. C. and G. K. Walker, 1993: A rain evaporation and
downdraft parameterization to complement a cumulus updraft
scheme and its evaluation using GATE data. Mon. Wea. Rev., 121,
3019-3039.
- Wu, X, J. J. Bates, and S. J. S. Khalsa 1993: A climatology of
the water vapor band brightness temperatures from NOAA
operational satellites. J. Climate, 6, 1282-1300.
Return to Eric Salathé,'s WWW Page